We already have the perfect energy storage – nuclear fuel
If decarbonizing global energy systems is a priority; it seems obvious that all low carbon options should be considered as part of the solution. Yet, a year and a half after 21 prominent scientists disproved the Jacobson paper that claimed a 100% renewables electricity system is feasible in the USA by 2050, it remains a challenge for many people to move on to more sensible solutions. Hence the Green New Deal that says this 100% renewable dream can not only be achieved, but in half the time. In reality, it still just won’t work.
Imagine a world where the electricity system works like the battery in your cell phone. You depend on your phone and worry the battery will run out just as you need it most. To make things worse, even though there is an electrical outlet available, you can’t charge your phone because these outlets don’t work all the time. To keep your anxiety in check, you must always carry spare batteries with you to make sure your phone doesn’t die at the least opportune moment. Assuming you make it through the day, you would like to charge your phone while you sleep so it is fully charged when you wake up ready for a new day. Unfortunately, you can’t charge it at night because your charger only works during the day at the same time you most use your phone. Planning to keep your phone charged becomes a constant pre-occupation as you go about your daily business.
This is the challenge with an electricity system based on variable intermittent renewable energy sources. We know that if we want to rely on wind and solar for all our electricity needs, that wind only produces about 30 to 40% of the time, and that solar panels only produce about 15% of the time. After all, we can’t make the sun shine, or the wind blow more than they do. Therefore, we need to find a way to save the energy produced when it is available using some type of storage – like the extra batteries for your cell phone – that will allow it to be used later when it is needed.
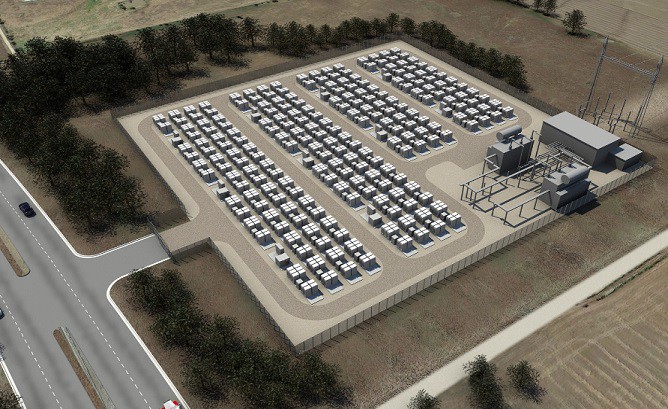
So how do you make sure you have enough energy to meet electricity demand reliably in this scenario? The only way would be to build lots and lots and lots of wind and solar, way more than you need at any given time and do your best to store this large amount of excess energy for later use. In other words, the intermittency of these resources means you have to build a HUGE amount of capacity coupled with a large amount of storage to get the same amount of energy you could otherwise get from a readily dispatchable resource that is available whenever you need it, (which is why the fossil industry loves this scenario because they know the most likely option is using gas plants to meet the demand when renewables cannot.)
An MIT study “The Future of Nuclear Energy in a Carbon – Constrained World” published last year looks at what is needed to fully decarbonize a system both with and without nuclear energy. As can be seen below, replacing fossil fuels without nuclear means having to build a system that is an ORDER OF MAGNITUDE larger that what is currently in place. Yes, that is right. Without nuclear, you need to build a system of renewable energy and storage that is on the order of 10 times larger than what you have in place today to try and make sure you will always have enough energy available to meet demand. After all, it would be hard to imagine a future where our economies accept that it’s OK to run out of energy until the next time the sun shines or the wind blows.
For example, as can be seen from the figure, to eliminate the emissions from a 500g/kWh system in New England without nuclear power would require increasing the size of the system from 35 GW to 286 GW to replace gas with renewables and storage. (About 500 g/kWh is an average carbon emission for many systems around the globe today. This study looks at what it would take to bring that close to zero.) The figure also shows that decarbonizing by replacing gas with a combination of nuclear and renewables (or “nuables”) results in a system with little change in size to what is in place today, and at much lower cost. (For New England, the cost would be about half of a fully renewable system.) The MIT study looks at many regions. Achieving the same result for the UK means increasing the system size from 58 to 478 GW while Zhejiang China would need to increase the size of its grid from 78 to 1515 GW to get off fossil fuels without using nuclear power.
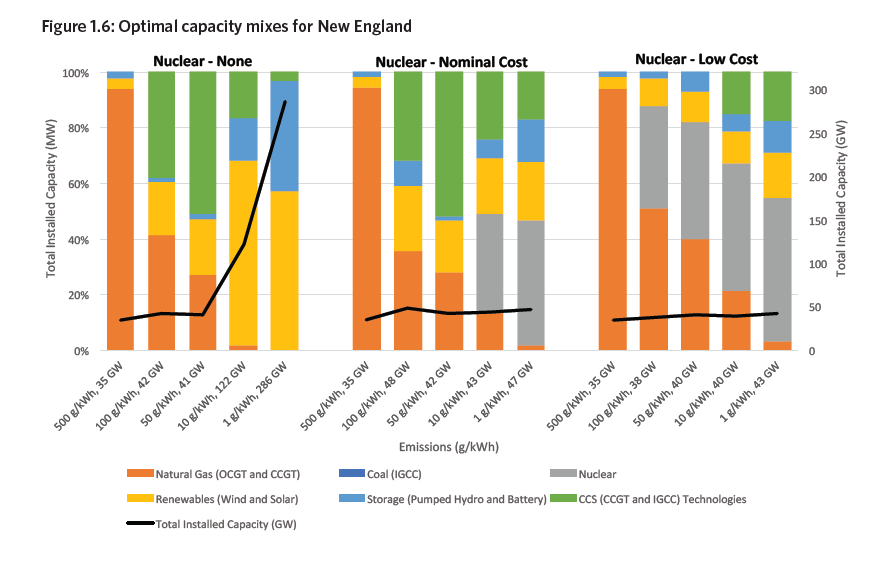
Source: MIT Study “The Future of Nuclear Energy in a Carbon – Constrained World”
We have seen this in action. To date as part of the Energiewende, Germany has doubled its system capacity to replace some of its nuclear with a massive amount of renewables all to deliver the same amount of energy to consumers with almost no impact to its carbon footprint, and at higher cost; all while still relying on coal as its most important form of generation.
This also bursts the fantasy that a fully renewable system is local and environmentally friendly as the electricity system (the grid) needs a huge amount of investment to support ten times as much capacity, not to mention the very large amounts of land needed to place these wind and solar collectors, and the huge amount of materials like steel and rare earths needed to build them and then all the waste when it comes time to dispose of them at their end of life.
As for storage, the task ahead is enormous. As stated in a recent article touting the benefits of battery storage from the IEA, “Today, pumped hydro storage systems account for the majority of storage capacity (153 GW, equivalent to about 2% of total power capacity worldwide, while battery storage systems total around 4 GW. However, while pumped hydro storage is projected to grow in the next decade, the technology deployment is largely constrained by the location of suitable sites.” This article then goes on to say battery storage can reach 400 to 500 GW by 2040, but this is still a drop in the bucket compared to what would be required. With the storage requirements for New England alone being about 100 GW, the global requirement would be in the many thousands of GWs to reach the levels required by a fully renewable system. And let’s not forget today’s batteries provide only short-term storage with technologies for long term storage nowhere near ready to meet a challenge of this magnitude.
Energy is most efficiently stored in fuel, like coal, gas or uranium, and then burned exactly when it is needed. And which fuel stores the most energy? Uranium. A single pellet of enriched nuclear fuel about the size of the end of your little finger, has the same amount of energy as one ton of coal. Or to put it another way, uranium produces about 3 million times more energy from a kg of U235 than coal does from a single kg of coal.
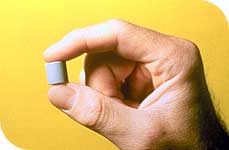
Now that is what I call energy storage. This little bit of fuel can produce a huge amount of energy and it is accessible to us when we want it. If we need to decarbonize our energy systems, and we want to do it relatively quickly, what makes more sense? Building a system that is ten times larger than we currently have to produce the same amount of energy we produce today, with all the materials and land that goes along with that, or building nuclear plants that can produce huge amounts of energy from a small amount of resource? I know which option would let me sleep at night – and would give me the best chance my phone alarm would actually work in the morning.
9 Comments
Paul Murphy · February 21, 2019 at 9:37 pm
Great analysis Milt
Sharon · February 21, 2019 at 9:59 pm
Most interesting blog, Milton.
Simple math in my mind,
Now if only the “”minds “” could figure it out
Ian Hore-Lacy · February 22, 2019 at 1:49 am
Also: OECD report highlights cost of intermittent renewables
A new report from the OECD’s Nuclear Energy Agency, The Costs of Decarbonization: System Costs with High Shares of Nuclear and Renewables, models different shares of nuclear power and renewables within a very tight 50g CO2 per kWh emission constraint. It quantifies the system costs – involved in delivering reliable power to meet demand – with different levels of variable renewable energy (VRE) input – solar and wind, and despite declining generation costs (and zero marginal costs) for those. The figures modelled are consistent with those now observable in some parts of the world. For instance, Germany has spent hundreds of billions on VRE over the last ten years while maintaining emissions at nearly ten times the above figure, but the delivered electricity cost has risen inexorably.
Since the load factor and the capacity credit of VRE sources is very much lower than that of conventional thermal or nuclear power plants, a significantly higher installed capacity is needed to produce the same amount of electricity. Modelling showed that installed capacity would need to more than double to achieve 50% VRE share, and more than triple for a 75% share, compared with fully-dispatchable base case, to meet the same demand. System costs rise accordingly, from less than $10/MWh for 10% VRE to more than $50/MWh at 75% VRE share. Of particular interest to countries such as Australia, without abundant hydro resources or interconnections to neighbouring countries, the system cost then approaches $50/MWh even at 50% VRE share. Hence at 75% VRE in the modelled case, or 50% in the case relevant to Australia, the delivered cost of electricity almost doubles from the fully-dispatchable base case with a large proportion of nuclear capacity. (In Germany, total installed capacity has more than doubled since 1990 to give only 19% more power with 24.6% share from wind+solar – which now represent half the total capacity – and electricity costs have risen about 50%.)
A striking effect of deploying a lot of wind and solar PV with low marginal generating cost is a substantial increase in the volatility of electricity prices, and at 30% VRE or above, zero prices sometimes occur, distressing financially to both VRE producers and the back-up reliable plants, and not compensated by the price peaks when VRE is insufficient. Since wind and solar PV output correlates with meteorological conditions across a wide area, increased VRE also means that the average price received by those producers – especially solar PV – declines significantly as their penetration increases. At a penetration level of 22.5%, the value of a megawatt-hour from wind is reduced by 25% in the model, and in Germany in 2018 the effect was even larger. Finally, and as strikingly shown in China in the last three years, high VRE means high curtailment rate on its output (up to 50% in some provinces in China).
OECD NEA http://www.oecd-nea.org/ndd/pubs/2019/7299-system-costs.pdf
Andrew Paterson · February 22, 2019 at 11:12 am
“URBAN DOMINANCE” and Nuclear Energy
Most of the nuclear capacity under construction today (60 GWs, says WNA, plus another 30 GWs with siting approved and final financing to be completed), is being driven by MASSIVE urbanization, mostly in Asia. Large modern cities (>1m people) cannot rely on VRE (wind and solar) for 24/7 loads to keep functioning with office towers, high rise apartments, mass transit, and more electric vehicles. Average usage statistics mask large regional differences within countries and fluctuations in VRE over time. Your article rightly points out the limits on storage, but batteries don’t do anything about the large swaths of land needed for wind and solar — in some places 10 or 20 times the area needed per MW compared to a nuclear plant even with a buffer zone. The energy density of nuclear without emissions is an obvious deal maker for nuclear in crowded Asia suffering severe levels of hazardous air pollution, but too often the large and steady emissions savings of nuclear is not reflected in prices; it is an externality to market pricing.
On emissions, witness that in Oct. 2018, James Hansen and many other scholars and scientists submitted an Open Letter to world leaders of the G20 declaring the importance of Nuclear Energy to address emissions related to Climate Change. Several of the world’s leading researchers on climate change signed this letter.
Open Letter to Heads of Government of the G-20 from Scientists and Scholars on Nuclear Energy for Climate Change [October 25, 2018]
http://environmentalprogress.org/big-news/2018/10/25/open-letter-to-heads-of-state-of-the-g-20-from-scientists-and-scholars-on-nuclear-for-climate-change
Unlike the UNFCCC, the G20 is a body with the financing capacity (and more than 70% of the world’s emissions and GDP) to seriously address de-carbonization.
Andrew Paterson, Environmental Business International
Duane Pendergast · February 22, 2019 at 12:58 pm
Great article Milt! I note that you are making the case for nuclear – even without dependency on arguments for atmospheric decarbonization. Humanity will need new sources of energy eventually and nuclear can provide it.
Janice Dunn Lee · February 23, 2019 at 10:03 am
Very compelling point Milt! Need to convince the new green dealers.
Canon Bryan · February 24, 2019 at 4:58 pm
What I don’t understand is how power systems using only renewables are rated at 1gCO2/kWh.
If solar life cycle emissions are around 50 g/kWh and wind life cycle emissions are around 12 g/kWh, how the hell do they get to 1 g/kWh?
I suspect the answer is that the analysis is a giant fraud — root and branch.
MIT certainly displayed extreme intellectual sloppiness in their assessment of the LCOE of innovative nuclear reactor designs. This shortcoming causes me question the scientific validity of the entire report.
Martin Kral · February 25, 2019 at 9:26 am
Speaking of stored energy, the US has over 100,000 tons of unused nuclear fuel rods and another several hundred thousand tons of stored deleted uranium (the world has even more). Now, all we need is a few thousand molten chloride salt fast reactors or equivalent to consume all that unused fuel for the next few centuries while electrifying the entire world without ever having to mine another gram of uranium.
As I sit here dreaming of how it could have been done by now, if and only if Dr. Alvin Weinberg (ORNL) had stood his ground back in the 1970’s with the Molten Salt Reactor Experiment. Instead, I have to experience the great Green New Deal future fiasco.
See my personal 50/50 Clean Energy Program (Part 1 and 2) at my blog (link below).
JoAnn Thompson · February 25, 2019 at 10:13 pm
Great article! Totally puts it in perspective.
Comments are closed.